The
flyback powered -
PLASMA
GLOBE!
[updated! +
Videos!]
 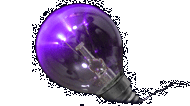 
Introduction: An overview on
what a plasma globe is and who invented it.
How it works: Basically, how it works.
Professional Globes: How they differ from mine!
My flyback driven plasma globe:
What and how I did it
Multimedia: More pictures and videos!
Plasma
globes (also known as plasma spheres, lightning globes, plasma lamps,
etc) were invented by Nikola Tesla, mainly for studying plasma.
You most
probably have seen them before. They are glass spheres with dancing
coloured streams of light and are often seen in science centres,
museums, sci-fi movies, science exhibitions, science fairs etc.. They
are very attractive, and different plasma globes have different
coloured streamers, some even with multiple coloured streamers.
Nowadays, one can purchase a professional plasma globe at a low cost.
On the right you can see a photo of a normal spherical plasma globe.
But how exactly
does a plasma globe works? In this page, I shall explain briefly how a
plasma globe work, how different effects can be achieved, and how I I
made my own plasma globe, including pictures and diagrams.
How does a
plasma globe work? Sam's Page on
Plasma Globes has a very nice
explanation which I quote:
"The
principle of operation in plasma globes involves two main concepts:
one is plasma dynamics, and the other one is capacitive current flow.
First let's look at what is inside the globe: The most obvious answer
would be "plasma"... But what really is plasma? Simply put, a plasma
is "a hot, ionized gas". It is also defined as being the 4th state of
matter, as it does not consist of molecules like the other 3 states:
Instead, a plasma is made up of ions. In order to understand how this
comes about, we have to look at what a plasma globe really is: A
middle electrode (sometimes insulated, other times not) sitting inside
a large vessel containing inert gas. The gas must be inert (I.E.
non-reactive) otherwise it will react with the electrode surface
(inert gases also have a low voltage breakdown). And it must be at low
pressure in order for a not-too-high voltage to be able to break it
down. Than the electrode is energized with a high voltage-high
frequency power supply. The high voltage breaks down the gas, and the
high frequency gets the current through the glass of the globe and
into the surrounding air by capacitive coupling. Typical voltages are
around a few thousand volts for most commercial plasma globes,
sometimes around 10,000 volts for some homebrew ones, or the larger
commercial ones. Typical frequencies are from a few to a few tens of
kilohertz.
Essentially, what is happening inside a plasma globe is the travel of
electrons from the electrode to the outer surface and an oscillating
electro-magnetic field. The motion of the electrons is necessary to
generate plasma. A plasma is simply a gas containing charged particles
such as electrons and ions. The electrons are broken free from a
parent atom or molecule, and that atom or molecule becomes an ion. The
electron has a negative charge, and the ion has a positive charge.
When these charged particles move about within the plasma, they are
changing the local characteristics of the electro-magnetic field. This
combined with the oscillating electro-magnetic field from the
electrode will "excite" ions, molecules, and atoms. When these
particles (whether they have a charge or not) become excited, they
very quickly radiate the energy in the form of a photon, or unit of
light. This is what makes the plasma emit it's characteristic color,
and the color will depend upon the gas that originated it, and it's
temperature. The characteristics of how these electro-magnetic fields
combine is what determines the overall appearance of the plasma globe.
The Electron
The electron will fly off of the electrode if the voltage at the
electrode is sufficiently high. What voltage is sufficiently high? The
very minimum voltage required for an electron to escape a metal
electrode is determined by the "work function" of the metal. The metal
electrode will hold the electron until the electron reaches a
potential sufficient to break this bond. For most metals, this is only
4 or 5 volts, and is called the work function. After the electron
leaves the electrode, ignoring the glass interface of the typical
plasma globe, the electron must then "collide" with and ionize an atom
or molecule before a plasma can be generated. There are many different
ionization states for the gases found in plasma globes, but the
important thing to know is that the electron must have a minimum
amount of energy to be able to ionize an atom or molecule, and this
energy is called "ionization potential". For gases such as nitrogen,
oxygen, neon, etc., the first ionization potential is typically 50 to
150 volts. So, for a plasma to be made, the electrode potential (i.e.
voltage) must be at least the sum of the first ionization potential of
your gas plus the work function of the electrode metal, and any extra
potential helps increase the plasma density.
"UNDER PRESSURE!"
Even though your plasma globe is "evacuated", it still has a HUGE
number of particles. A typical 8" diameter plasma globe (with a
pressure of ~500 milliTorr) will still have 100 billion billion
particles! Assuming all of the particles are spaced evenly, this means
the average distance between particles is so small that approximately
2500 particles span a length equal to the diameter of a human hair!
So, an electron does not need to travel very far to "interact" with an
atom or molecule. In fact, the mean free path of the molecules
themselves is about half of a millimeter. An electron can travel
farther than this without interacting with a molecule, but not much
farther, due to to electromagnetic interactions (as opposed to
collisions, which have a rather long mean free path).
The pressure in the globe will help determine the characteristics of
the streamers. The pressure in a plasma globe is actually much higher
than the pressure found in most plasma chambers used for scientific
experiments. In fact, the pressure needs to be this high to see the
streamers. The pressure is so high that when plasma is generated, it
actually gets hot. Since hot air rises, the streamers will tend to
move up the side of the globe. Also, this hotter region has much
higher conductivity, since it is a plasma, and therefore the streamers
remain intact until an instability breaks them. This is the same
principle behind a Jacob's ladder (the cool looking arc traveling up
between two wires commonly seen in Frankenstein movies - it gets
longer as it goes up the wires). If the pressure in a globe is too
high, the potential of the electrode will not be sufficient for the
electrons to generate a plasma, and you will see nothing. If the
pressure is too low, the effects of the "fluid" nature of the
streamers will be gone - and you will only see an overall illumination
of the globe, with no streamers. The intensity of this light will be
rather low, also.
"I am beginning to see the light!"
So, how is the light generated? Now we are getting into the physics of
atoms and molecules, which involves the gory details of quantum
mechanics.
The effect that causes an ion, molecule, or atom to release light is
called spontaneous emission. This occurs when a particle (read
particle as ion, atom, or molecule) is in an "excited" state. This
basically means that the particle has more energy than a stable
particle, so it is unstable. The particle will spontaneously emit a
photon (a unit, or "quanta" of light), which reduces the particles
energy to a more stable condition, or "state". This photon is visible
only if it has an optical wavelength. Most of the excitation states
for particles that will emit light are such that the wavelength of the
emitted light is visible, making plasma easy to see if the density is
sufficiently high. Also, these emitted photons are of an exact
wavelength. Each time a particle goes from the same higher energy
state to the same lower energy state, the same photon will be emitted.
This is what gives gases their characteristic appearance - only
certain colors are capable of being emitted. The ONLY way to change
the color of the plasma is to change the gases inside the chamber, or
increase the electrode voltage to increase the number of energy states
possible (and therefore emission states, however, when you do this,
the plasma color usually tends towards white or blue).
So what causes a particle to become "excited"? No innuendoes here, but
particles in a plasma globe are easily excited by a charged particle
flying sufficiently nearby if the (moving) particle has enough energy.
Either an electron or ion can serve as the charged particle - but
generally this occurs due to an electron-particle interaction. The
electron will lose some energy by transferring it to the particle. The
particle then releases this energy in the form of a photon. You can
see the light emitted only if thousands of particles are
"spontaneously emitting" light at essentially the same time - simply
to get an intensity high enough for the human eye to detect. In a
plasma globe, billions of such interactions are occurring.
Putting it all together
For extremely energetic electrons (voltages at least 10 times the
first ionization potential) many interactions can occur before the
electron loses it capability to ionize or excite particles in the
globe. Such high potentials are typically not needed for a scientific
plasma, since pressures are low and methods of confining the plasma
are heavily used. In a globe, the plasma particle has an extremely
short life (before it becomes a neutral particle again) due to the
higher pressure and lack of magnetic confinement.
Plasma displays, such as the very common Eye of the Storm displays,
typically have only one electrode from which plasma trails or streams
propagate. This type of IGDT (Inert Gas Discharge Tube) requires a
very high voltage, high frequency AC power supply. Since the IGDT has
only one electrode, the return path for the current flowing inside the
tube is the air itself. The capacitance between the high voltage
electrode and the circuit ground is the only return path. Current must
flow via this stray capacitance through the surrounding air to the
circuit ground. The stray capacitance is quite low which is why the
voltage and frequency must be so high. The path that the plasma trails
follow varies a lot during operation for several reasons.
The plasma trails created in the IGDT tend to move rather randomly and
are generally dimmer and thinner than plasma flowing through
sign-tubes, which have two electrodes. The trails keep moving because
the charged or ionized gas areas keep moving. Charge builds up in
areas without trails until they ionize. Then the charge carriers in a
cloud region collapse into an ion trail, which is a good conductor,
which allows current to flow to circuit ground, draining the charge.
Once the region is discharged, the trail may disappear or migrate in
some direction towards another area, which is charged. The trail will
continue to exist as long as sufficient current can flow. You yourself
can become a return path by touching the glass surface because you are
a better conductor than the surrounding air. One very important thing
to consider is that since the power supply is operating at a high
frequency, the plasma globe or tube is in fact an antenna. You are
like an antenna in many respects as well. High frequencies cause a
skin effect, which prevents you from receiving a shock. The currents
you carry are very low and tend to flow along your surfaces. The glass
envelope and the plasma trail itself are also providing decoupling
from the power supply electrode. This protects you to some degree.
Since the display globe acts like an antenna, it conducts or transmits
more power near its self-resonant frequency. It will have the most
trails when operating at that frequency because the power transfer is
most efficient. The oscillating field that is generated can transmit
power into and through other objects, which come into the field. It is
very easy to make other tubes, especially Neon and florescent ones,
glow in your hand just by holding them inside the electromagnetic
field near the operating plasma globe. You can then use a simple Neon
candle-flicker bulb to observe the relative field strength at any
point in space surrounding the display because the bulb will light up
accordingly as the field excites the gas within.
Power supplies designed for plasma displays can also drive neon sign
tubes. If you want to do this, you should connect only one electrode
and leave the other electrode disconnected and insulated. If you
connect both electrodes, the current that flows through the tube can
be very high. At the high frequency at which a plasma power supply
operates, the thin Neon tube's electrodes may concentrate the current
towards the metal surfaces of the electrodes. This skin effect can
increase the apparent impedance or resistance of the electrode and
cause a significant (exponential) rise in power dissipation &
therefore they'll run a lot hotter than they were designed to.
When current flows, people have described electrons moving in one
direction and positive ions moving the other way. This in fact occurs
in certain circuits, which rely on the electrochemical transfer of
atoms of an electrode through an electrolyte material. This process
occurs in batteries. This does not occur in IGDTs or typical
semiconductor circuits. While the electrons do in fact move from atom
to atom, the atoms themselves pretty much remain where they are. Light
is emitted when an atom loses an electron, thereby changing to a lower
energy level. This happens to the atoms in the slurry of gas millions
and millions of times per second as the electrons make their way along
the plasma trail. This means they are constantly changing their state
of charge relative to their neighbors and they'll just bounce around
willy-nilly all over the place. As a result, the positive ions do not
remain positive ions for long. Even if they did and even though it is
true that positive ions would be slightly attracted to a negatively
charged electrode at one end, they really don't move much because the
physical forces of pressure continuously act to keep the gas evenly
distributed throughout the tube. Some people call the places left
behind when an electron leaves an atomic orbit a hole oddly enough,
which technically makes the atom a positive ion. It is said that the
holes move one way while electrons move the opposite way. Holes are
not actually things or particles as electrons are so even though both
statements made about what is moving is technically true, I prefer to
say the electrons are moving rather than the absence of them or the
nothingness. In either case, the atoms themselves pretty much stay
put. Proof of this is simple to observe. Just look at the light
emitted in a normal florescent tube. Pretty evenly distributed isnt
it?"
Information above quoted under permission from Sam Barros'
PowerLabs,
http://www.powerlabs.org
Today, are are many different
kinds of plasma globes. The most common ones uses a spherical glass
globe, filled with a specialized low-pressure gas mix. Because
professional plasma globes use a very low pressure, they usually have
lower voltages and use a lower powered power supply. The different
mixture of gasses give the globes different colours (from helium to
neon, to krypton etc) . Furthermore, the lower power allows the globe
to be safely touched, unlike mine which has too high a current to
handle safely.
As you can see in the pictures
below, globes come in all sorts of shapes and sizes.
Constructing a plasma globe like
professional globes is difficult. A strong glass globe is needed, as
well as a powerful vacuum pump and special gases to fill the globes.
As such, I have a different approach.
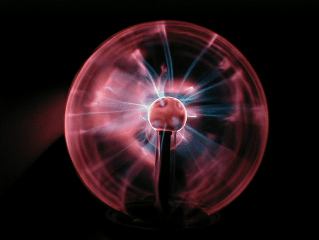 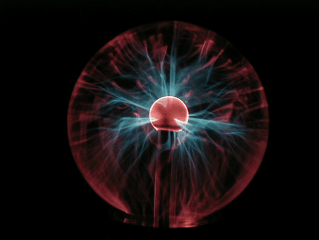
Beautiful
'Eye of the storm' globes
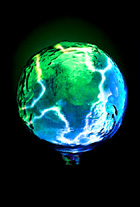 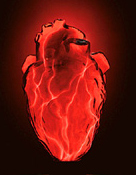 \
Globes
come in all sorts of shapes and colours nowadays!
To
your right you can see a picture of the setup from above. This
experiment was performed by a lighting transformer flyback driver
instead of the solid state transistorized version. I used a cheap
Electronic Halogen Lighting Transformer because it was sufficient and
easier to put together than the transistorized version. Also, I did
not have a decent power supply for driving the transistor circuit. View my other
flyback page on how to build a driver for it.
*Update 23 March 04! I have
acquired a new 34A 12VDC power supply. The transistor version is now
working. More powerful arcs and voltages can be achieved! More updates
to come!
Here, you can see my entire
setup. The connector connects the mains to the transformer to the
flyback. The primary is 13 turns of insulated copper wire at 11.5V,
20khz AC. It is a good non-rectified flyback and produces large long
arcs. The backdrop is black to facilitate easy photo taking and I will
be using 40W (big fat one) and 60W (thinner bottomed one) light bulbs
for the experiment. (Apparently, they don't make much of a
difference). The copper bar acts as a grounded object for the arcs to
arc to in the globe. Click to see a full view.
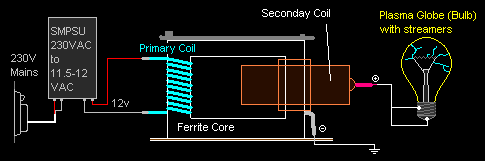
And here's a
diagram of how I wired it all up. Quite simple actually.
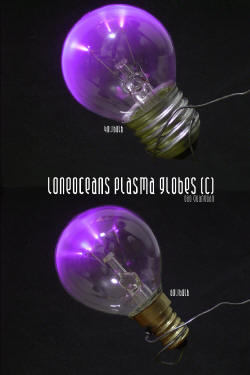
This 2 pictures show show a ~4cm
diameter 40W and 60W incandescent globes attached to the driver. A
word of warning: the plasma streams are extremely tightly focused and
they can carry a lot of current (lethal currents, if your power supply
is not limited). This is typical of Nitrogen. You'll notice that the
streams in a light bulb have the very familiar lightning-like
appearance. Our air is almost 80% Nitrogen hence the similarity.
Furthermore, UV light is produced, harmful in large amounts.
As you can see, the plasma
streams are going in an upward direction due to the electrodes and
also the rising action of the hot plasma! Touching it gives a tingling
effect to the fingers. Do not touch it! By putting a sharp metal point
on the surface makes the plasma streams extremely focused and at a
slightly higher level, it would melt and burn through the glass! From the
pictures, the two different bulbs don't have much of a difference
though.
Click the picture for a full
view.
(Actually my camera doesn't get
the correct colours. Only the colour of these images have been edited
to look more like the actual colours. The photo come out looking
bluish instead of purplish.)
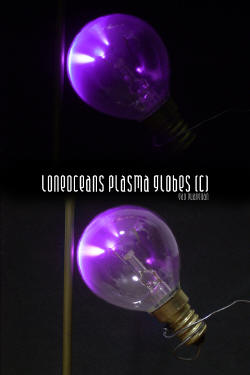
Here to the right you can
see see the streamers trying to arc to the copper rod which is
grounded. Notice how intense the streamers are. This hot plasma
channel forms when I touch it too. You can't touch it longer for a few
seconds before it gets really hot, enough to give a nice burn.
The plasma fills up to bulb with a nice purple glow.
In real life the arcs are bight
purple, some almost white.
Click Images to view in full.
[Update! More Pictures!]
With no more bulbs lying around
at home, I went to the mart and purchased a cheap 100W clear
incandescent bulb for 50c. It's cheap, clear and quite large compared
to my 40 and 60W bulbs. Anyway, I connected it and fired it up.
Instead of the normal purplish arcs, some arcs were quite red,
possibly due to slightly different gas mixtures inside the glass
envelope, like argon.
Here you can see the streamers arcing to the metal pole in a 1/2
second exposure. Notice the banjo effect as the streamers rise up
(they are hot, and therefore rises) . Also notice the other corona breakouts along
the sharp points in the bulb.
These
two are one of my favourite shots. It's 1/42 second exposure with the
flash. Nice reddish to purple streamers can be see breaking out from
two sharp points.
An interesting shot. The streamer
is almost all red,...
Fierce arcing to the metal rod can be observed.
The streamers formed within the
100W bulb were longer and more spectacular. Some streamers turned out
reddish which produced marvelous colours. Remember to look at the
videos section.
Download the last video the see this bulb in action!
[More Photos!]
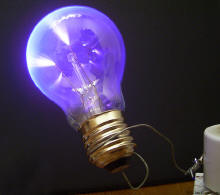 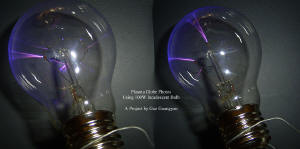
A marvelous display of the
larger 100W bulb (6cm dia) with beautiful streamers. The first one is
a 1sec exposure picture showing the bright purple streamers. The next
two pictures on the right show how wonderful the streamers are (1/52
sec exposure). Look at those colours! Most of the steamers beak out
from two main points.
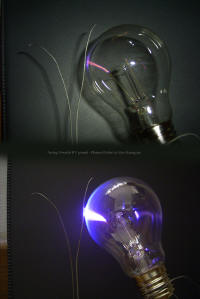
Here you can see two pictures
(one with flash and one longer exposure) of arcs from the plasma globe
towards HV ground. Again, a nice reddish arc can be seen in the first
picture. I am particularly fond of these nice red arcs which blend
into purple. You can also make out tiny arcs from the glass towards
the HV ground.
Not only can does this flyback
power bulbs, photoflash xenon tubes work as well!
They light up extremely
brightly. Bright white!
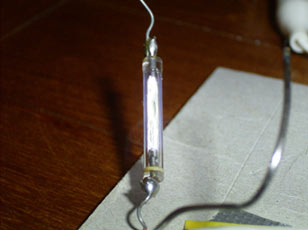
What lovely pictures..! I am now
looking for other bulbs such as neon bulbs or such for better plasma
displays.
Other
Information
Here are some links to other
pages which are very useful:
> Wikipedia:
Plasma Globes:
http://en.wikipedia.org/wiki/Plasma_globe
> Sam's
Powerlabs Page on Plasma Globes:
http://www.powerlabs.org/plasmaglobes.htm# TECHNICAL%20DETAILS
> Plasma
Spheres, Lightning Globes:
http://members.misty.com/don/plaspher.html
> Bill's
Science Hobbyist Plasma Globe Page:
http://www.amasci.com/tesla/heli1.html
Here are some clips of the
plasma globe in action!
(you need Windows Media Player to view the videos which are encoded in
WMV format)
Plasma globe in action / Arcing
to a grounded object
plasma.wmv [310kb]
[update!]
Exciting Plasma Bulb collection video!
bulb.wmv [830kb]
[Note:
The arcs in real life are purple in colour, much like the picture
below which has been digitally recoloured]
Your simple home made plasma globe! |